NAD+ (nicotinamide adenine dinucleotide)
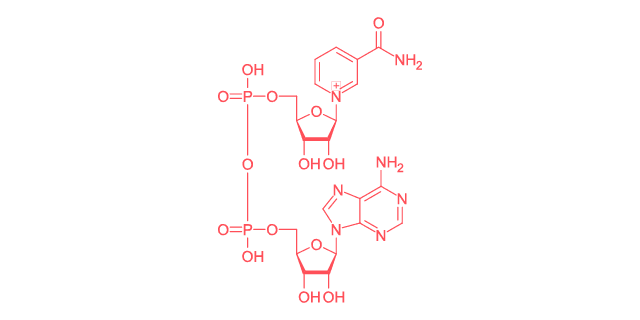
Linear Formula
C21H27N7O14P2
Synonyms
NAD; Adenine dinucleotide, dihydronicotinamide; nicotinamide-adenine-dinucleotide
Share this metabolite
Nicotinamide adenine dinucleotide (NAD+) is a co-enzyme synthesized from dietary tryptophan via the kynurenine pathway. NAD+ homeostasis in mammalian bodies is maintained through the salvage pathway, where NAD+ is recycled from nicotinamide and other NAD+-related molecules. It plays a vital role in regulating redox reactions, glycolysis, cellular metabolism, and metabolic pathways, specifically acting as a redox carrier where it receives a hydrogen ion from several metabolic processes to form NADH. Mitochondrial NADH then participates in ATP synthesis and generation of reactive oxygen species (ROS).1 Recent findings have since expanded the roles of NAD+ to include DNA repair, modulation of inflammatory pathways, circadian rhythm, stress responses, and other critical cellular processes.
Given the diverse impact NAD+ has on multiple major biological processes, emerging reports have implicated NAD+ pathways in many diseases. NAD+ deficiency has been linked to metabolic disorders, cancer, and age-related diseases, including degenerative brain diseases and disorders. Since the primary source of NAD+ comes from the intake of NAD+ dietary precursors like tryptophan (often from cheese, meats, nuts, etc.) or supplements that promote NAD+ generation (e.g., Vitamin B3), considerable research has been dedicated to understanding NAD+ deficiency and restoring it through diet or supplementation.
NAD+ and Metabolic Health
Impairments in glucose metabolism characterize metabolic disorders like obesity and type-2 diabetes, and NAD+ deficiency has been observed in animal models of metabolic dysfunction and patients with obesity or type-2 diabetes. For instance, due to impaired β-cell function in pancreatic islets, elevated insulin levels result in a failure of glucose to initiate an increase in NADH and ATP, ultimately resulting in severe deficits in energy metabolism and mitochondrial function.2 Other reports have demonstrated that NAD+ levels are linked with insulin resistance: knockout of NAMPT, a critical gene for NAD+ synthesis, results in marked insulin resistance in mice that can be rescued by administration of a NAD+ intermediate, nicotinamide mononucleotide (NMN).3
Similarly, NAD+ levels in many tissues, including skeletal muscle, brain, liver, and adipose tissue, are significantly reduced in obesity.4 For example, overexpression of microRNA-34a, a gene regulator that is elevated in obesity, reduces NAD+ levels and results in obesogenic phenotypes in mice.5 These phenotypes include increased expression of inflammatory markers, increased lipogenesis, elevated ER-stress, and decreased fatty-acid β-oxidation.
NAD+ and Gastrointestinal Health
The interaction between gastrointestinal health and the gut microbiome has gained considerable interest for its impact on overall human health, and emerging research has begun to link NAD+ as a vital modulator of gut microbiome function. Interestingly, recent studies performed in germ-free mice revealed a robust decrease in NADH/NAD+ ratio,6 and germ-free mice fed a high-fat diet exhibit increased liver NAD+.7 These results suggest that NAD+ and the gut microbiome interact to regulate critical metabolic processes.
Dietary intake of NAD+ precursors is absorbed in the proximal gastrointestinal tract, and microbes in the distal gut cannot access these precursors. Instead, circulating nicotinamide enters the gut lumen, where gut microbes convert nicotinamide to nicotinic acid. It is then utilized in host tissues to support NAD+ synthesis.8 While the contribution of NAD+ and microbiome interactions to metabolic dysfunction is still under investigation, these results suggest that this process might be critical for the flexible regulation of energy production and NAD+ homeostasis.
NAD+ and Cancer
In addition to its role as a co-enzyme that modulates metabolic redox reactions, NAD+ also impacts the development of tumorigenesis and governs genome stability, cell growth and death, and immune responses. The growth of cancer cells is metabolically demanding, resulting in the reprogramming of resources to accommodate energy requirements.
While healthy cells’ mitochondria effectively convert NAD+ to NADH by oxidative phosphorylation, NADH in cancer cells is insufficient to meet energy demands. Consequently, alternative pathways (e.g., oncogenic receptor tyrosine kinase FGFR1 by lactate dehydrogenase) are activated to promote increased NADH/NAD+ ratios, thereby facilitating tumor growth.9 This excess metabolism leads to heightened production of ROS that promotes genomic instability and cell death.10 Other reports have emphasized the importance of NADH/NAD+ homeostasis, where changes in either direction can profoundly impact cancer development. For example, enhancement of mitochondrial complex I, a critical regulator of NADH/NAD+ ratios, inhibits tumor growth in breast cancer cells. On the other hand, reduction of NAD+ levels resulted in more aggressive tumor growth.11
Cancer is often characterized by genomic instability, DNA repair failure, and altered transcriptional patterns. In response to genomic instability in tumors, poly(ADP-ribose) polymerases (PARPs; human PARP protein family) are deployed for repair, and PARP inhibitors are commonly used in cancer treatments. Significantly, PARPs heavily rely on NAD+ to repair DNA lesions. Recent evidence shows that individuals with ovarian cancer and high levels of the NAD+ derivative, NADP+, are more sensitive to PARP inhibitors.12 Collectively, these data provide a novel biomarker for PARP inhibitor treatment and implicate a role for NAD+ as a tool for determining treatment responses in cancer patients.
NAD+ and Neuroscience
Age-related decline in cognitive and motor functions is a hallmark of many neurodegenerative diseases. NAD+ deficiency has been shown to accelerate aging processes and has been implicated in several degenerative brain diseases, including Alzheimer’s disease, Parkinson’s disease, Huntington’s disease, and amyotrophic lateral sclerosis (ALS).1 Indeed, NAD+ is vital for energy maintenance in the brain and promotes neuronal morphogenesis. NAD+ deficiency is prevalent across many degenerative neurological diseases and disorders.
Recent evidence suggests that Alzheimer’s disease is partly characterized by oxidative damage and genomic instability. Interestingly, in mouse models of Alzheimer’s disease, restoring NAD+ levels with nicotinamide riboside significantly improved cognitive decline, reduced DNA damage, and decreased hippocampal apoptosis.13 Individuals with Parkinson’s disease also exhibit NAD+ deficiency, mitochondrial dysfunction, and oxidative stress. Notably, NAD+ supplementation in mouse models of Parkinson’s disease using NAD+ precursors (e.g., nicotinamide riboside) or enhancement of NAD+ salvage pathways have been shown to protect against mitochondrial defects and prevent cell death.14
NAD+ and Drug Development
NAD+’s widespread and often beneficial effects have opened avenues for this compound as a potential therapeutic.15 As described, NAD+ and NAD+ precursor supplementation often exert therapeutic and protective effects against aging, metabolic dysfunction, cancer, and degenerative neurological diseases. Nicotinamide riboside (i.e., vitamin B3) has been examined as a supplement to protect against aging-related diseases and is generally considered safe by the FDA.16 Studies have also begun to investigate the efficacy of NAD+ upregulation to enhance immune cell activation in viral infections, including COVID-19.17 However, elevating NAD+ levels as a therapeutic remains under investigation.18
NAD+ and Research
As of August 2023, there are over 140,000 citations for “nicotinamide adenine dinucleotide” and “NAD+” in research publications (excluding books and documents) on Pubmed. The vast number of publications linking this molecule and its metabolites to a broad range of physiological functions and diseases suggests that any research program seeking to understand better metabolic disorders, cancer, aging, and degenerative diseases and disorders of the brain and nervous system may benefit from quantitative analysis of NAD+ and NAD+ precursors. Preclinical research may also benefit from NAD+ quantification for a global understanding of biomarkers, diagnosis, and disease monitoring.
References
- Xie, N, Zhang, L, Gao, W, et al. NAD(+) metabolism: pathophysiologic mechanisms and therapeutic potential. Signal Transduct Target Ther 2020;(5):227.
- Haythorne, E, Rohm, M, van de Bunt, M, et al. Diabetes causes marked inhibition of mitochondrial metabolism in pancreatic beta-cells. Nat Commun 2019;(10):2474.
- Stromsdorfer, KL, Yamaguchi, S, Yoon, MJ, et al. NAMPT-Mediated NAD(+) Biosynthesis in Adipocytes Regulates Adipose Tissue Function and Multi-organ Insulin Sensitivity in Mice. Cell Rep 2016;(16):1851-1860.
- Yoshino, J, Mills, KF, Yoon, MJ, et al. Nicotinamide mononucleotide, a key NAD(+) intermediate, treats the pathophysiology of diet- and age-induced diabetes in mice. Cell Metab 2011;(14):528-536.
- Choi, SE, Fu, T, Seok, S, et al. Elevated microRNA-34a in obesity reduces NAD+ levels and SIRT1 activity by directly targeting NAMPT. Aging Cell 2013;(12):1062-1072.
- Donohoe, DR, Garge, N, Zhang, X, et al. The microbiome and butyrate regulate energy metabolism and autophagy in the mammalian colon. Cell Metab 2011;(13):517-526.
- Backhed, F, Manchester, JK, Semenkovich, CF, et al. Mechanisms underlying the resistance to diet-induced obesity in germ-free mice. Proc Natl Acad Sci U S A 2007;(104):979-984.
- Chellappa, K, McReynolds, MR, Lu, W, et al. NAD precursors cycle between host tissues and the gut microbiome. Cell Metab 2022;(34):1947-1959 e1945.
- Fan, J, Hitosugi, T, Chung, TW, et al. Tyrosine phosphorylation of lactate dehydrogenase A is important for NADH/NAD(+) redox homeostasis in cancer cells. Mol Cell Biol 2011;(31):4938-4950.
- Cairns, RA, Harris, IS, and Mak, TW. Regulation of cancer cell metabolism. Nat Rev Cancer 2011;(11):85-95.
- Santidrian, AF, Matsuno-Yagi, A, Ritland, M, et al. Mitochondrial complex I activity and NAD+/NADH balance regulate breast cancer progression. J Clin Invest 2013;(123):1068-1081.
- Bian, C, Zhang, C, Luo, T, et al. NADP(+) is an endogenous PARP inhibitor in DNA damage response and tumor suppression. Nat Commun 2019;(10):693.
- Hou, Y, Lautrup, S, Cordonnier, S, et al. NAD(+) supplementation normalizes key Alzheimer’s features and DNA damage responses in a new AD mouse model with introduced DNA repair deficiency. Proc Natl Acad Sci U S A 2018;(115):E1876-E1885.
- Lehmann, S, Loh, SH, and Martins, LM. Enhancing NAD(+) salvage metabolism is neuroprotective in a PINK1 model of Parkinson’s disease. Biol Open 2017;(6):141-147.
- Arenas-Jal, M, Sune-Negre, JM, and Garcia-Montoya, E. Therapeutic potential of nicotinamide adenine dinucleotide (NAD). Eur J Pharmacol 2020;(879):173158.
- B. Poljsak, IM. Vitamin B3 forms as precursors to NAD+: Are they safe? Trends in Food Science & Technology 2018;(79):198-203.
- Omran, HM, and Almaliki, MS. Influence of NAD+ as an aging-related immunomodulator on COVID-19 infection: A hypothesis. J Infect Public Health 2020;(13):1196-1201.
- Radenkovic, D, Reason, and Verdin, E. Clinical Evidence for Targeting NAD Therapeutically. Pharmaceuticals (Basel) 2020;(13).